Metamaterials: All You Need to Know
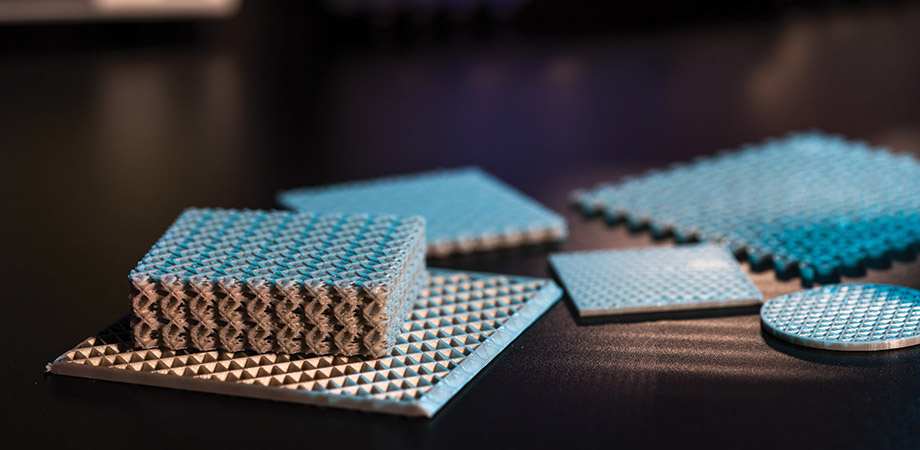
Metamaterials represent a class of artificially engineered materials designed to exhibit properties not found in naturally occurring substances. They are meticulously crafted at a scale smaller than the wavelength of the phenomena they influence, allowing for precise manipulation of electromagnetic, acoustic, mechanical, or thermal waves. Unlike conventional materials, which derive their properties from their chemical composition and atomic structure, metamaterials derive their unique characteristics from their precisely designed internal structure. Let’s dive deep into metamaterials and learn their properties, applications, and many more.
Historical Background of Metamaterials
The concept of metamaterials traces back to the early 20th century, with the theoretical groundwork laid by scientists such as Victor Veselago in the 1960s. Veselago’s pioneering work proposed the idea of materials exhibiting a negative refractive index, which initially met with skepticism due to its departure from classical physics principles. However, materials science and nanotechnology advancements in the late 20th and early 21st centuries paved the way for the realization of metamaterials.
Importance of Metamaterials in Modern Science and Engineering
The development of metamaterials has significantly impacted various fields of science and engineering, offering unprecedented control over the behavior of waves and enabling revolutionary applications. Some key areas where metamaterials have made substantial contributions include:
- Electromagnetic Wave Manipulation: Metamaterials have enabled the realization of concepts once considered purely theoretical, such as invisibility cloaks and superlenses. By manipulating the propagation of electromagnetic waves, metamaterials have opened new avenues in optics, telecommunications, and information technology.
- Acoustic and Mechanical Wave Control: Acoustic metamaterials have revolutionized the control of sound waves, leading to applications in noise reduction, acoustic cloaking, and vibration damping. Similarly, mechanical metamaterials have demonstrated unprecedented mechanical properties, offering opportunities for lightweight yet strong structural materials with tailored mechanical behaviors.
- Thermal Management: Metamaterials have also shown promise in the field of thermal management, with applications ranging from thermoelectric energy conversion to thermal insulation and heat transfer control. By engineering materials’ thermal conductivity and emissivity at the nanoscale, metamaterials can improve energy efficiency and thermal comfort in various applications.
Fundamental Principles of Metamaterials
1. Manipulating Electromagnetic Waves
Metamaterials are renowned for their ability to manipulate electromagnetic waves in unconventional ways, enabling a range of groundbreaking applications in optics, telecommunications, and beyond. This manipulation is achieved through the precise arrangement of subwavelength structures within the metamaterial, which interact with incident electromagnetic waves at the nanoscale.
2. Subwavelength Scale and Structured Materials
At the heart of metamaterial design lies the concept of subwavelength scale, wherein the components of the metamaterial are smaller than the wavelength of the waves they interact with. This subwavelength scale allows for precise control over the behavior of electromagnetic waves, overcoming the limitations imposed by natural materials.
Metamaterials typically comprise structured materials engineered at the nanoscale, including metallic elements such as gold or silver, dielectric materials like silicon dioxide, and artificially synthesized nanostructures. These structured materials are arranged in patterns to achieve desired electromagnetic properties, such as negative refractive index or anomalous dispersion.
3. Key Properties: Negative Refractive Index, Dispersion, and Anisotropy
Negative Refractive Index: One of the defining properties of metamaterials is their ability to exhibit a negative refractive index, a phenomenon not found in naturally occurring materials. In conventional materials, light bends away from the normal when entering a medium with a higher refractive index. However, light bends in the opposite direction in metamaterials with a negative refractive index, leading to unique optical behaviors such as reverse refraction and subwavelength imaging.
Dispersion: Metamaterials also exhibit anomalous dispersion, where the phase velocity of light is not proportional to its frequency as in natural materials. This anomalous dispersion allows for manipulating light propagation in ways impossible with conventional materials, enabling applications such as superlens, where subwavelength imaging is achieved using metamaterial-based lenses.
Anisotropy: Another characteristic of metamaterials is their anisotropic nature, meaning their electromagnetic properties vary depending on the direction of wave propagation. This anisotropy arises from the asymmetric arrangement of subwavelength structures within the metamaterial, allowing for directional control of electromagnetic waves. Anisotropic metamaterials find applications in devices such as polarizers, waveguides, and beam-steering antennas.
Types of Metamaterials
Metamaterials represent a diverse class of engineered materials designed to manipulate waves in various electromagnetic, acoustic, mechanical, and thermal domains. Each type of metamaterial exhibits unique properties and functionalities tailored to specific applications, ranging from optics and telecommunications to structural engineering and thermal management.
1. Electromagnetic Metamaterials
Electromagnetic metamaterials focus on manipulating electromagnetic waves, particularly in the optical and microwave frequency ranges. Electromagnetic metamaterials can achieve extraordinary optical properties such as negative refractive index, perfect absorption, and anomalous dispersion by arranging subwavelength structures at the nanoscale. These properties enable various applications, including superlenses for subwavelength imaging, invisibility cloaks for electromagnetic concealment, and metasurfaces for controlling light polarization and phase.
2. Acoustic Metamaterials
Acoustic metamaterials are designed to control the propagation of sound waves in various environments, offering unprecedented noise control, vibration damping, and acoustic cloaking capabilities. By structuring materials at the subwavelength scale, acoustic metamaterials can manipulate the transmission, reflection, and absorption of sound waves with high precision. Applications of acoustic metamaterials include soundproofing materials for architectural and industrial noise reduction, acoustic filters for frequency-selective attenuation, and metamaterial-based devices for directing and focusing sound waves in underwater communication systems.
3. Mechanical Metamaterials
Mechanical metamaterials focus on manipulating mechanical waves, such as elastic waves and vibrations, in structural and mechanical systems. Mechanical metamaterials can achieve extraordinary mechanical properties, including negative stiffness, wave propagation control, and topological protection by engineering the geometry and arrangement of subwavelength structures. These properties enable innovative applications in structural engineering, mechanical damping, and vibration isolation, offering lightweight and resilient materials for aerospace, automotive, and civil engineering applications.
4. Thermal Metamaterials
Thermal metamaterials are designed to control the flow of heat and thermal energy, offering unique capabilities in thermal insulation, heat transfer modulation, and energy conversion. By structuring materials at the nanoscale, thermal metamaterials can accurately manipulate the thermal conductivity, emissivity, and diffusivity. Applications of thermal metamaterials include thermal insulators for building and automotive applications, thermoelectric materials for energy harvesting and waste heat recovery, and radiative cooling materials for passive cooling systems in arid climates.
Harnessing Sound with Acoustic Metamaterials
Acoustic Cloaking and Invisibility
Acoustic metamaterials have revolutionized the control of sound waves, enabling the development of acoustic cloaking devices that render objects invisible to sound. By engineering the acoustic properties of metamaterials at the subwavelength scale, it’s possible to bend and redirect sound waves around an object, effectively cloaking it from detection. Applications of acoustic cloaking include stealth technology, architectural noise reduction, and underwater stealth for submarines and marine vessels.
Acoustic Filters and Wave Manipulation
Acoustic metamaterials also find applications in developing acoustic filters and wave manipulation devices. By tailoring the geometry and arrangement of subwavelength structures, metamaterials can selectively filter specific frequencies of sound waves while allowing others to pass through. This capability enables the development of acoustic lenses, waveguides, and beam-steering devices for directing and focusing sound waves in various applications, including medical imaging, sonar systems, and ultrasonic therapy.
Soundproofing and Noise Control Applications
One of the primary applications of acoustic metamaterials is soundproofing and noise control in architectural, industrial, and transportation settings. Metamaterial-based soundproofing materials can attenuate unwanted noise by absorbing, reflecting, or scattering sound waves. These materials find applications in building construction, automotive engineering, and aerospace design to mitigate noise pollution and improve acoustic comfort in indoor and outdoor environments.
Applications of Metamaterials in Optics
High-Resolution Imaging and Microscopy
Metamaterials have revolutionized the field of optics, enabling high-resolution imaging and microscopy beyond the diffraction limit. Superlenses constructed from metamaterials with negative refractive index can focus light at subwavelength scales, allowing for the visualization of nanostructures and biological molecules with unprecedented detail. Metamaterial-based microscopy techniques hold promise for advancing biomedical research, nanotechnology, and materials science.
Photonic Crystals and Bandgap Engineering
Metamaterials play a crucial role in the design and fabrication of photonic crystals, periodic structures that control the propagation of light waves. By engineering the photonic bandgap of metamaterial-based photonic crystals, it’s possible to manipulate the flow of light and achieve unprecedented control over its properties. Photonic crystals find applications in optical communication, optical computing, and photonic integrated circuits for next-generation information technologies.
Metamaterials for Enhanced Light-Matter Interaction
Metamaterials have also enhanced light-matter interaction in various systems, enabling enhanced spectroscopy, nonlinear optics, and plasmonic sensing applications. By tailoring the electromagnetic properties of metamaterials at the nanoscale, it’s possible to enhance light absorption, emission, and scattering in nanophotonic devices and sensors. Metamaterial-based optical devices are promising for biosensing, environmental monitoring, and photovoltaics applications.
Metamaterials in Aerospace and Defense
Radar and Stealth Technology
Metamaterials have revolutionized radar and stealth technology by enabling the development of stealth aircraft and radar-absorbing materials (RAMs). Metamaterial-based RAMs are engineered to absorb and scatter electromagnetic waves, reducing aircraft’s radar cross-section and making them less detectable by enemy radar systems. These materials find applications in military aircraft, naval vessels, and unmanned aerial vehicles (UAVs) for stealth operations and enhanced survivability in combat scenarios.
Antenna Miniaturization and Efficiency
Metamaterials play a crucial role in antenna miniaturization and efficiency enhancement in aerospace and defense applications. Metamaterial-based antennas can achieve compact designs and improved performance compared to traditional antennas, making them ideal for integration into small-scale platforms such as UAVs, satellites, and wearable communication devices. Metamaterial antennas offer size, weight, and power consumption advantages, enabling advanced communication and surveillance capabilities in constrained environments.
Metamaterials for Advanced Sensors and Imaging Systems
Metamaterials find applications in advanced sensors and imaging systems for aerospace and defense applications, enabling enhanced detection, tracking, and reconnaissance capabilities. Metamaterial-based sensor arrays and imaging systems leverage the unique electromagnetic properties of metamaterials to achieve high-resolution imaging, multispectral sensing, and electromagnetic wave manipulation for target identification and threat assessment in military and surveillance operations.
Medical and Healthcare Applications of Metamaterials
Biomedical Imaging and Diagnostic Tools
Metamaterials have transformative applications in biomedical imaging and diagnostic tools, offering enhanced non-invasive imaging and disease detection capabilities. Metamaterial-based imaging techniques, such as metamaterial-enhanced magnetic resonance imaging (MRI) and terahertz imaging, enable high-resolution visualization of biological tissues and organs with improved sensitivity and specificity. These imaging modalities hold promise for early detection and diagnosis of various medical conditions, including cancer, cardiovascular diseases, and neurological disorders.
Therapeutic Devices and Drug Delivery Systems
Metamaterials find applications in developing therapeutic devices and drug delivery systems for targeted treatment of diseases and disorders. Metamaterial-based devices, such as implantable sensors and drug-eluting implants, enable precise control over drug release and therapeutic interventions, improving treatment outcomes and patient care. Metamaterials also have the potential for innovative therapeutic modalities, such as hyperthermia and photodynamic therapy, for cancer treatment and tissue regeneration.
Wearable Metamaterial-based Health Monitoring Devices
Metamaterials are integral to developing wearable health monitoring devices that enable real-time monitoring of vital signs and physiological parameters for personalized healthcare. Metamaterial-based sensors and wearable devices offer non-invasive and continuous monitoring of parameters such as heart rate, blood pressure, and glucose levels, facilitating early detection of health issues and timely intervention. These wearable devices promise to improve patient outcomes, enhance remote patient monitoring, and enable proactive management of chronic diseases.
Challenges and Future Directions
Fabrication Techniques and Scalability
Despite significant progress in metamaterial research, challenges remain in developing cost-effective and scalable fabrication techniques for large-scale production. Current fabrication methods often involve complex and time-consuming processes, limiting the scalability of metamaterials for commercial applications.
Dynamic Tunability and Reconfigurability
Another challenge in metamaterial research is achieving real-time dynamic tunability and reconfigurability of metamaterial properties. While static metamaterial designs offer precise control over specific wave phenomena, dynamic control of metamaterial properties is essential for adaptive applications such as reconfigurable antennas, tunable optical devices, and dynamically modulated sensors. Future research efforts focus on incorporating active materials and external stimuli-responsive mechanisms to achieve dynamic tunability in metamaterials.
Integration with Existing Technologies and Commercialization
Integrating metamaterials with existing technologies and commercialization presents compatibility, performance optimization, and market adoption challenges. Metamaterial-based devices and systems must demonstrate compatibility with conventional technologies and seamless integration into existing infrastructure to realize their full potential. Furthermore, addressing regulatory and standardization requirements is crucial for commercializing metamaterial-based products and fostering market acceptance across industries.
Conclusion
Metamaterials represent a groundbreaking technology with transformative potential across various scientific and technological fields. Through ongoing research and innovation in metamaterial design and fabrication, we stand on the cusp of revolutionary breakthroughs in telecommunications, healthcare, renewable energy, and quantum computing. These materials offer unprecedented opportunities for ultrafast communication systems, advanced medical imaging, efficient solar energy harvesting, and quantum information processing.
However, unlocking the full potential of metamaterials requires sustained research efforts and collaborative initiatives among scientists, engineers, and industry stakeholders. Investment in research infrastructure, interdisciplinary collaboration, and knowledge exchange are essential to overcome existing challenges and drive metamaterial advancements. With continued progress, metamaterials are poised to become a cornerstone of next-generation technologies, addressing global challenges and fostering economic growth while shaping a future of limitless possibilities.
0 Comments