Solid-state Batteries: Everything You Need to Know
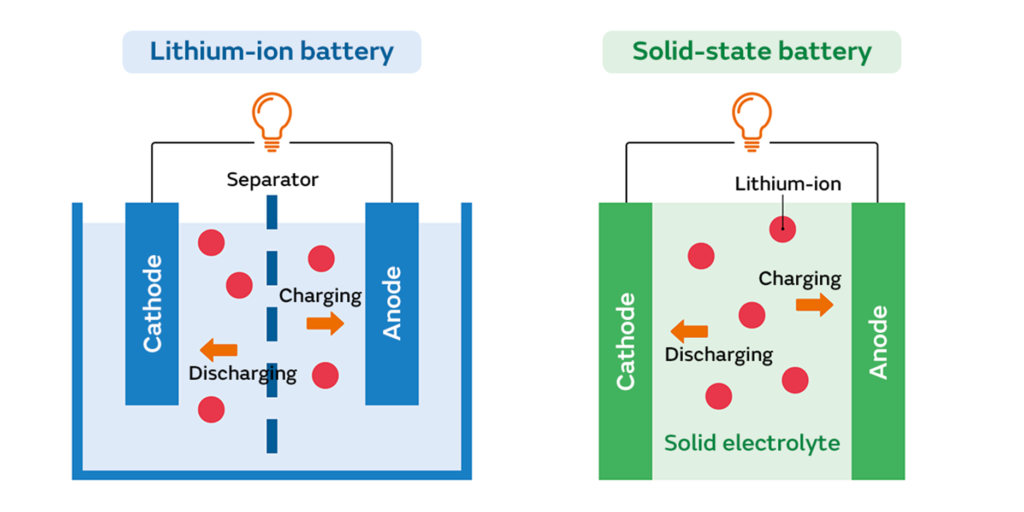
Solid-state batteries represent a revolutionary advancement in energy storage technology, offering a promising alternative to traditional liquid electrolyte-based batteries. Unlike conventional batteries, which utilize liquid or gel electrolytes, solid-state batteries employ solid electrolytes to transport ions between the cathode and anode.
Solid-state batteries have three primary components: the cathode, anode, and solid electrolyte. The solid electrolyte serves as a medium for the transport of ions (typically lithium ions in lithium-ion batteries) between the cathode and anode during charge and discharge cycles. This solid-state architecture eliminates the need for flammable liquid electrolytes, enhancing safety and stability.
Solid-state batteries have gained significant attention due to their potential to address key limitations of conventional batteries, including safety concerns, energy density, and cycle life. By replacing liquid electrolytes with solid-state materials, these batteries offer improved thermal stability, reduced risk of leakage or explosion, and enhanced energy density and lifespan performance. Let’s dive deep into this technology.
Importance of Energy Storage in Modern Society
Energy storage plays a crucial role in modern society, serving as a cornerstone for various sectors, including transportation, renewable energy integration, grid stability, and portable electronics. The increasing demand for reliable and sustainable energy solutions has propelled the need for efficient energy storage technologies.
In transportation, energy storage systems are vital for electrifying vehicles, including electric cars, buses, and trains. Battery technology enables the storage of electricity from renewable energy sources such as solar and wind, facilitating their integration into the power grid and reducing dependence on fossil fuels.
Moreover, energy storage solutions contribute to grid stability by providing backup power during peak demand periods, minimizing grid fluctuations, and enabling efficient load management. This capability is particularly crucial with the growing penetration of intermittent renewable energy sources.
In portable electronics such as smartphones, laptops, and wearables, energy storage plays a pivotal role in powering devices and enabling mobility. Consumers demand lightweight, compact, and long-lasting batteries to support their increasingly mobile lifestyles.
Given these diverse applications and the evolving energy landscape, advancements in energy storage technology, including solid-state batteries, are essential for addressing current challenges and advancing toward a more sustainable and resilient energy future.
Transition from Conventional Batteries to Solid-State Batteries
The transition from conventional to solid-state batteries represents a significant paradigm shift in energy storage technology. Traditional lithium-ion batteries, while widely used, face inherent limitations related to safety, energy density, and environmental impact.
Solid-state batteries offer a compelling alternative by addressing these limitations through their unique design and materials. The use of solid electrolytes eliminates the risk of electrolyte leakage, thermal runaway, and fire hazards associated with liquid electrolytes, enhancing overall safety.
Furthermore, solid-state batteries exhibit higher energy densities than conventional batteries, enabling increased energy storage capacity within the same footprint. This advancement is crucial for applications requiring compact and lightweight energy storage solutions, such as electric vehicles and portable electronics.
Additionally, solid-state batteries demonstrate superior cycle life and stability, promising longer-lasting and more durable energy storage systems. This attribute is precious for applications with critical battery longevity and reliability, such as grid-scale energy storage and mission-critical devices.
The transition from conventional to solid-state batteries represents a transformative shift towards safer, more efficient, and sustainable energy storage solutions. Continued research and development efforts in this field hold the potential to accelerate the adoption of solid-state batteries across various industries, driving innovation and addressing pressing energy challenges.
Structure and Composition of Solid-State Batteries
Solid-state batteries comprise several key components that store and release energy. These components include:
- Solid Electrolyte: The solid electrolyte is a critical component of solid-state batteries, serving as the medium through which ions (e.g., lithium ions) travel between the cathode and anode during charge and discharge cycles. Solid electrolytes replace the liquid electrolytes found in conventional batteries and are typically made from various solid materials with high ionic conductivity.
- Anode: The anode is the electrode where oxidation (release of electrons) occurs during battery discharge. In solid-state batteries, the anode material is typically a lithium-containing compound, such as lithium metal, lithium titanium oxide, or lithium iron phosphate. The choice of anode material impacts battery performance, including energy density, cycle life, and stability.
- Cathode: The cathode is the electrode where reduction (acceptance of electrons) occurs during battery discharge. Similar to the anode, the cathode material influences battery performance parameters. Common cathode materials used in solid-state batteries include lithium cobalt oxide, lithium manganese oxide, lithium nickel manganese cobalt oxide (NMC), and lithium iron phosphate.
- Current Collectors: Current collectors are conductive materials that facilitate the flow of electrons between the electrodes and the external circuit. In solid-state batteries, current collectors are typically made from metals such as aluminum or copper and are coated with active materials (anode or cathode materials) to enhance conductivity and stability.
- Separator: The separator is a porous membrane that physically separates the cathode and anode while allowing the transport of ions between them. In solid-state batteries, separators are often made from ceramic or polymer materials with high ionic conductivity and mechanical strength to withstand the operating conditions.
- Packaging and Housing: Solid-state batteries are enclosed within a protective housing or packaging to ensure safety and integrity. The packaging materials must be chemically inert, electrically insulating, and mechanically robust to prevent leakage, short circuits, and external damage.
Solid Electrolytes: Types and Characteristics
Solid electrolytes are a key component of solid-state batteries, providing a stable and conductive medium for ion transport between the cathode and anode. Several types of solid electrolytes are used in solid-state batteries, each with unique characteristics and properties:
- Inorganic Solid Electrolytes: Inorganic solid electrolytes are typically ceramics or glass ceramics composed of elements such as lithium, sulfur, phosphorus, and oxygen. Examples include lithium phosphorus oxynitride (LiPON), lithium garnet (e.g., Li7La3Zr2O12 or LLZO), and sulfide-based electrolytes. Inorganic solid electrolytes offer high ionic conductivity and excellent chemical stability at room temperature but may suffer from brittleness and processing challenges.
- Polymer Solid Electrolytes: Polymer solid electrolytes consist of organic polymers doped with lithium salts to enhance ionic conductivity. Common polymer electrolytes include polyethylene oxide (PEO), polyvinylidene fluoride (PVDF), and polyethylene oxide-based copolymers. Polymer electrolytes offer flexibility, ease of processing, and mechanical robustness but may exhibit lower ionic conductivity and stability than inorganic electrolytes.
- Composite Solid Electrolytes: Composite solid electrolytes combine inorganic and polymer components to synergize their advantages and mitigate their limitations. Examples include ceramic-polymer and polymer-ceramic composites, which offer improved mechanical strength, flexibility, and ionic conductivity compared to pure polymer or ceramic electrolytes.
Characteristics of solid electrolytes include high ionic conductivity, chemical stability, mechanical strength, and compatibility with electrode materials. The choice of a solid electrolyte depends on factors such as battery performance requirements, manufacturing considerations, and application-specific constraints.
Anode and Cathode Materials in Solid-State Batteries
Anode and cathode materials are crucial in determining solid-state batteries’ performance, energy density, and stability. Various materials are used for the anode and cathode in solid-state batteries, each with unique properties:
Anode Materials:
- Lithium Metal: Pure lithium metal offers the highest theoretical specific capacity and energy density but is prone to dendrite formation, which can cause short circuits and reduce battery lifespan.
- Lithium Titanate (Li4Ti5O12): Lithium titanate is a spinel-structured material known for its high rate capability, excellent cycle life, and low risk of lithium dendrite formation. However, it has lower specific capacity compared to lithium metal.
- Lithium Iron Phosphate (LiFePO4): Lithium iron phosphate is a cathode material known for its high stability, safety, and long cycle life. It exhibits lower specific capacity than lithium metal but improves safety and durability.
Cathode Materials:
- Lithium Cobalt Oxide (LiCoO2): Lithium cobalt oxide is a widely used cathode material known for its high specific capacity and energy density. However, it has cost, safety, and cobalt availability limitations.
- Lithium Nickel Manganese Cobalt Oxide (NMC): NMC is a ternary cathode material composed of nickel, manganese, and cobalt. It offers a balanced combination of specific capacity, voltage, and cycle life, making it suitable for various applications.
- Lithium Iron Phosphate (LiFePO4): As mentioned earlier, lithium iron phosphate is not only used as a cathode material but also as an anode material in some solid-state battery configurations. Its high stability, safety, and long cycle life make it a preferred choice for certain applications.
Anode and cathode materials selection depends on factors such as desired energy density, cycle life, safety requirements, cost considerations, and application-specific needs. Researchers continue to explore new materials and electrode architectures to enhance further the performance and efficiency of solid-state batteries for diverse applications.
Working Principles of Solid-State Batteries
Solid-state batteries operate on the same basic principles as conventional batteries but with a different architecture. Instead of using liquid electrolytes, solid-state batteries employ solid electrolytes, separators, and electrodes. This solid-state architecture offers several advantages over traditional batteries, including enhanced safety, higher energy density, longer lifespan, and improved environmental sustainability.
Ion Transport Mechanisms
In solid-state batteries, ion transport occurs through the solid electrolyte material rather than through a liquid electrolyte. Various mechanisms, including ionic diffusion and migration through the solid electrolyte lattice can facilitate this. Solid electrolytes typically used in solid-state batteries include ceramic materials (e.g., lithium phosphorous oxynitride, lithium garnet), polymer electrolytes, and glass electrolytes.
Electrochemical Reactions in Solid-State Batteries
Solid-state batteries undergo electrochemical reactions similar to conventional batteries, involving the movement of ions between the electrodes through the solid electrolyte. During charging, ions migrate from the positive electrode (anode) to the negative electrode (cathode), and during discharging, ions move back to the anode. The reactions at the electrodes depend on the specific chemistry of the battery, such as lithium-ion, sodium-ion, or solid-state lithium-sulfur batteries.
Impact of Operating Conditions on Performance
Various operating conditions, including temperature, current density, cycling rate, and state of charge influence the performance of solid-state batteries. High temperatures can accelerate degradation, while low temperatures can reduce ion mobility and decrease performance. Additionally, high current densities and rapid cycling rates can lead to mechanical stress and degradation of the electrodes and electrolyte, affecting battery performance and lifespan.
Advantages of Solid-State Batteries
- Enhanced Safety Features: Solid-state batteries are inherently safer than conventional lithium-ion batteries due to the absence of flammable liquid electrolytes, reducing the risk of thermal runaway and fire hazards.
- Higher Energy Density: Solid-state batteries have the potential for higher energy density than conventional batteries, enabling longer driving ranges for electric vehicles and increased energy storage capacity for portable electronics.
- Longer Lifespan: Solid-state batteries typically exhibit longer cycle life and calendar life than traditional batteries, reducing the need for frequent replacements and maintenance.
- Environmental Sustainability: Solid-state batteries often utilize more environmentally friendly materials and manufacturing processes than conventional batteries, reducing the environmental impact of battery production and disposal.
Challenges and Limitations
- Manufacturing Complexity: Solid-state batteries require specialized manufacturing processes and equipment due to the use of solid electrolytes, which can increase production costs and complexity.
- Cost Considerations: The current cost of solid-state batteries is higher than conventional lithium-ion batteries, primarily due to the manufacturing complexity and the use of expensive materials.
- Scalability Issues: Scaling up the production of solid-state batteries to meet commercial demand remains a challenge due to manufacturing complexities and the need for further research and development to optimize production processes.
- Performance Degradation over Cycles: Solid-state batteries may experience performance degradation over cycles, including capacity fade and impedance growth, which can impact overall battery performance and lifespan.
Conclusion
In conclusion, solid-state batteries offer a transformative solution across various sectors. With enhanced safety, higher energy density, longer lifespan, and environmental sustainability, they address critical needs in the automotive, consumer electronics, renewable energy, and aerospace/defense industries.
Solid-state batteries promise extended driving ranges and faster charging for electric vehicles in automotive. In consumer electronics, they enable smaller, safer devices with longer battery life. Renewable energy support grid stability and reduces environmental impact. In aerospace/defense, they offer lightweight, safe, and reliable energy solutions.
Despite challenges, ongoing research aims to overcome limitations. With continued investment, solid-state batteries are set to revolutionize energy storage and drive a sustainable future.
0 Comments